The Path to Prevention: Dissecting Aging through Proteomics
- Vahik Soghom
- Sep 28, 2023
- 13 min read
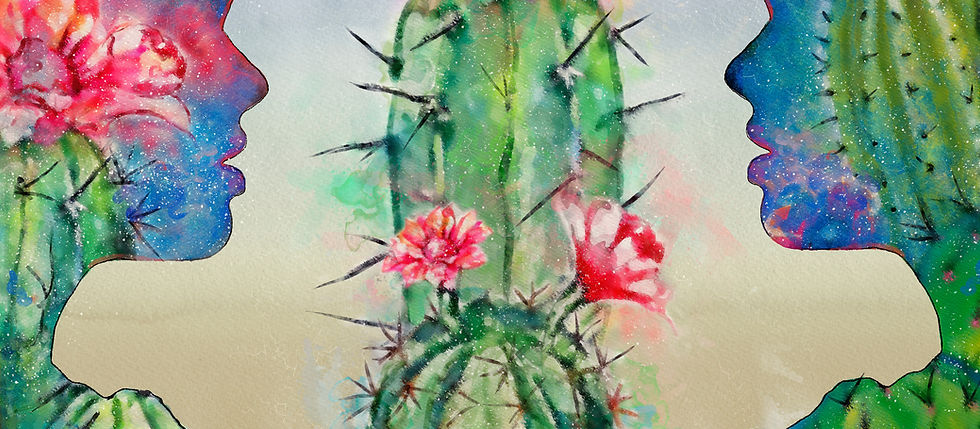
“I wish that your limbs were as supple and your strength as sure as your judgment is; but age, the common enemy of mankind, has laid his hand upon you; would that it had fallen upon some other, and that you were still young.”
Agamemnon to Nestor, The Iliad, Book IV
Aging is the leading cause of disease and disability, and has been viewed as such as early as Homer wrote on the Trojan war.
Every human living till old age will ultimately succumb to the “common enemy of mankind”. Yet aging is highly variable and complex. The Gods must have favored Nestor by only taking away his physical vigor. Whereas most mortals experience the full wrath of age by falling victim to vastly different yet debilitating and life-threatening diseases.
We can, however, forgive Homer for his fatalistic take on old age. After all, it took three thousand years from the end of the Bronze Age for life expectancy to take great leaps forward - due, in part, to medical breakthroughs that led to better care.
Yet lifespan extension has not been met with a commensurate extension of healthspan. Between 2000 and 2019, life expectancy increased by 6 years globally, and though health expectancy increased by 8%, this was due to declining mortality and not to fewer years living with disability.1 People may be living longer but they’re also suffering longer. And a globally increasing older population will only exacerbate both individual suffering and societal pain, through gargantuan expenditures on elderly care and immense psychological care burdens on friends and relatives.
We have therefore transitioned from a society facing acute illness to one dealing with complex age-related chronic illness. And our healthcare system, being fundamentally based on detecting and managing disease, is not designed to effectively prevent and treat chronic diseases.
For healthspan to catch up with lifespan, it’s incumbent upon us to view aging itself as a disease. And to treat this complex process that unfolds over a lifetime, we need a deeper understanding of aging to develop diagnostic and therapeutic tools that prevent or delay age-related disease onset. In essence, we need to identify and validate early aging biomarkers that can inform novel preventative interventions.
The good news is that scientists, non-profits, and precision medicine companies across the globe are conducting groundbreaking research into aging and campaigning for regulatory reform to classify aging as a disease.
While regulatory reform could have a major impact on society as a whole, it’s important to recognize that the research landscape is still young and fragmented. But over the past decade, one field of research that has shown real promise is the development of aging biomarkers (also known as aging clocks or molecular aging clocks, henceforth molecular clocks). Molecular clocks try to estimate the organism’s biological age by looking at molecular changes in the body to predict and prevent health risks. The promise of this work is that in having tools that measure health status and not just identify the presence of disease, we will be able to detect risks early and develop validated preventative interventions.
As we’ll cover in more detail below, there are several approaches to building molecular clocks. Some choose to look at the epigenome as the seat of these molecular changes, while others look at the metabolome, glycome, transcriptome, or proteome. Molecular changes abound in all of these layers, and the goal is to uncover unique aging biomarkers that can inform preventative diagnostics and personalized interventions.
Teal is an early-stage startup focused on bringing innovative science to this field. Building on findings from their founding team's research at Stanford University, Teal uses human proteomics to develop a proprietary platform that estimates biological age and for the discovery and development of novel drug targets and biomarkers. Like other companies in our space, we founded Teal with the belief that by bringing strong science to the longevity field, we have an opportunity to accelerate the development of new treatments and solutions for extending healthspan and lifespan.
Molecular Aging Clocks: an Early Peek into Aging
Aging is a complex process with heterogeneous outcomes. It unfolds constantly throughout life and manifests differently in different people. Since their inception in 2011, molecular clocks have shown promise as novel tools to dissect, measure, and monitor aging in ways that capture this complexity and variability between individuals.
These clocks exploit the intuitive distinction between one’s chronological age as indexed by date of birth, and biological age, the organism’s age as expressed by molecular changes in the body. Simply put, if John’s biological age is 50 while he’s really 48, John is an accelerated ager, and vice-versa.
By looking closely at the dynamic biological changes that occur with age and their association with clinical outcomes, molecular clocks can offer an early view into aging and even predict health risks. One question that is still open for debate is: what makes a good clock? Current differences primarily lie in which layer of molecular markers they choose to investigate - the epigenome, transcriptome, proteome, metabolome, glycome, or microbiome.
The motivation to look at molecular markers to understand aging stems intuitively from our current healthcare system, where blood circulating molecules like insulin and CRP are measured to identify disease. “Identify” is the key word here. With our current technology, we are limited to looking too late, because disease is already present, and thus our solutions and interventions have naturally focused on disease management and reversal. Molecular clocks open the possibility of looking at earlier molecular changes that precede disease and lead to it. Age-associated diseases progress slowly, and with the right biological information we can be better at targeting causes of disease rather than symptoms.
Since the first seminal papers in the field, researchers have devised dozens of molecular clocks using different omics modalities. The earliest and most notable are epigenetic clocks, pioneered by Steve Horvath2 3 and Gregory Hannum4. These clocks look at DNA methylation - a mechanism used by cells to control gene expression - to determine biological age.
These first-generation epigenetic clocks were trained to predict chronological age based on DNA methylation levels at select CpG sites, but discrepancies between actual chronological age and predicted age outputs raised the question of whether DNA methylation might be useful in tracking the biological age of the organism. The next generation of clocks trained their models on indirect clinical biomarkers to predict biological age. Levine et al.’s DNAm PhenoAge clock, for instance, used nine such biomarkers, including white blood cell count, creatinine, albumin, glucose, and others in an attempt to correlate these outcomes with DNA methylation levels.5 Horvath’s GrimAge clock selected further biomarkers like leptin, cystatin C, growth differentiation factor, and more.6 Finally, the latest clocks, notably the DunedinPace clock, train DNA methylation data on more direct hallmarks of aging like grip strength, brain shrinkage, dental health, lung function, etc.7
If DNA methylation can be correlated to these hallmarks of aging, the biological age outputs of these models can thus serve as a baseline for identifying faster and slower agers and their corresponding mortality and morbidity risks.
As one would expect, DNAm PhenoAge and DNAm GrimAge show improved associations with mortality and morbidity risk as compared to the first-generation Horvath and Hannum clocks. Intriguingly, the DunedinPage clock appears to perform even better at assessing these risks.
The Virtues of a Good Molecular Clock
We can clearly see why epigenetic clocks are popular and a continuing subject of research. They’ve been shown to serve as a reliable predictor of mortality risk and give consistent outputs across tissue and cell types as well as across species. In addition to this, rejuvenation interventions have even been shown to turn back the epigenetic clock. Perhaps epigenetic clocks have a place in the future of clinical diagnostics?
But the story doesn’t end with epigenetic clocks. We believe there is room to improve our understanding and measurements of biological age in ways that offer better value for preventive diagnoses and precision medicine.
Ultimately, what makes a clock a better clock is the extent to which it displays two virtues we consider essential:
A good clock should be interpretable by offering insights into the underlying biology driving aging-related changes. Second, it should be actionable by providing the relevant information that directs that individual to the right intervention. A clock that has these virtues is one that adds to our understanding of aging and its underlying biological processes. It’s also a good candidate for delivering high-utility insights for the development of clinical diagnostics and therapeutics.
With that said, it’s clear that the epigenetic story isn’t there yet.
Let’s start with interpretability. The epigenetic story is limited by a relatively poor understanding of the underlying biology driving age-related changes.8 9 What initiates epigenetic changes and how are they involved in disease etiology? Much more work needs to be done to answer this question. In the meantime, a lack of mechanistic understanding limits the interpretability of epigenetic clocks.
Epigenetic clocks can also be noisy, sometimes leading to vastly different estimates of biological age when assessing the same sample.10 Moreover, several studies have shown no or inconsistent associations between epigenetic clock outputs and several aging phenotypes. For instance, GrimAge showed accelerated biological aging after physical activity as opposed to an expected decrease in biological age.11 And while the GrimAge clock was associated with a decline in physical functioning in women in a three-year follow-up study, other epigenetic clocks showed no association, and chronological age alone provided similar estimates of declining physical functioning.12 These findings show that epigenetic clocks are not as interpretable as one might wish them to be.
Another issue worth mentioning is that epigenetic clocks relate to some known aging hallmarks but not others. A study by Ken Raj and Steve Horvath showed that while epigenetic clocks relate to nutrient sensing, mitochondrial activity, and stem cell composition, they don’t relate to cellular senescence, genomic instability, and telomere attrition.13 While it’s unfair to expect any molecular clock to relate to all known disease biology, this mixed picture does add a further layer to the interpretability problem.
The question of correlation vs. causation should also be considered here. Do we believe that DNA methylation is a cause of aging or just a biological response?
The jury is still out, even if we consider evidence that rejuvenation interventions can set back the epigenetic clock. Several studies14 have shown that cell reprogramming using induced pluripotent stem cells (iPSCs) can alleviate age-related symptoms and lower the epigenetic age of cells.15 Whether this shows that DNA methylation is causally constitutive of aging remains unclear.
Let’s move on to actionability. A good clock should be able to inform personalized interventions. Unfortunately, the interpretability problem has a limiting impact here too. How can a robust personalized intervention plan be implemented if epigenetic clocks are not highly interpretable? Furthermore, epigenetic clocks are generally tasked with inferring the broad health status of an individual from biological information from a single tissue source. We know that epigenetic signatures vary across the body so we are limited in how granular we can get in our understanding of the heterogeneity of aging. Longevity interventions may indeed set back the epigenetic clock, but we may be limited in our ability to use this data to guide precision medicine.
To illustrate this point, let’s take an admittedly simplistic but poignant example.
Imagine that John undergoes multiple checkups at his local hospital, including an epigenetic test to estimate his biological age. Results show his epigenetic age is shown to be 50, although he’s actually 48. How can Dr. Smith interpret this information? She can certainly infer that John has a higher risk of mortality and morbidity within the next five to ten years. But that’s not enough to drive targeted interventions. So she tells him to sleep and eat well, exercise, not smoke, and come back in 6 months for another checkup. Pretty good, but not good enough.
Teal’s Proteomics Platform Unlocks New Possibilities
So what would be good enough? To paint a picture before diving deeper into the science, let’s assume that Dr. Smith uses Teal’s test to determine John’s health status.
Instead of a single biological age output, Dr. Smith can review the health status (“age”) of each organ system, each providing critical information into John's specific risks. Her attention is drawn to John's brain, which is 2 years older than his chronological age. She knows that accelerated brain age has been linked to increased Alzheimer's risk and the key area for her to focus John’s prevention program. Most of his other organs are in line with his chronological age. Surprisingly, his kidney is even younger.
John is a brain ager. While he has no other symptoms, our platform tells Dr. Smith that he’s at a higher risk of several degenerative brain conditions over the next 15 years. With this information, she can make targeted recommendations that might help John prevent disease altogether, or at the very least delay symptom onset. Indeed, our platform recommends two dietary supplements Teal has found to measurably improve brain age and reduce the risk of these degenerative conditions. These can be safely administered to John and are backed by Teal’s scientific studies. John feels optimistic about his chances.
Teal’s platform delivers on both key virtues:
Interpretability: The biological age outputs of John's organs are interpretable. His brain is older than his chronological age, which means he's likely to suffer from a brain-related disease. Other age-related diseases aren't ruled out, of course, but Dr. Smith has reliable information to stratify John’s health risk and to prioritize his care.
Actionability: Dr. Smith can take action to prevent or delay disease onset. Not only does she have recourse to a set of general interventions that are likely to help John address his accelerated brain aging, Teal's platform offers her two dietary supplement options that have been shown to reduce brain age and help combat disease onset.
Ultimately, Teal's platform is more useful to Dr. Smith. It enables her to peek into John's current health status in more detail while making reliable estimates about future health risks and ultimately recommending personalized interventions.
Now to the science. What drives our platform's unique ability to deliver better clocks that are interpretable, actionable, and - ultimately - more impactful?
The first answer has to do with the proteome itself. Protein functions are well-studied and characterized. They have direct functional impact by carrying out cellular functions and executing instructions from the genome and epigenome. Changes in protein expression, structure, and function are thus more proximal to functional aspects of aging. The proteome is also embedded with tissue-specific information with high biomarker potential, as evidenced by the common use of protein biomarkers in clinical diagnostics. They're also common drug targets, and are thus perfect candidates for targeted intervention.
The second answer has to do with Teal's domain expertise. Teal is a spinoff of the Wyss-Coray lab at Stanford. Teal’s Scientific Founder, Dr. Tony Wyss-Coray, has been a pioneer in the longevity space for over 20 years. His work to explore the role of proteins in neurodegenerative diseases has led to groundbreaking discoveries, specifically as it relates to the rejuvenating impact of blood plasma. In his renowned 2014 study, Tony and his colleagues showed that blood plasma from younger mice improved cognition and synaptic plasticity in older mice after connecting their circulatory systems.16 This phenomenon - known as heterochronic parabiosis - continues to be studied as a major prospective longevity intervention, the key hypothesis being that the proteome is likely central to rejuvenation effects. Teal’s approach is built around the belief that the proteome is tightly linked to the aging process, if not causally constitutive thereof.
The third - and related - answer has to do with Teal’s proprietary analytics approach. Protein markers have been shown across numerous studies to be useful in determining biological age. However, Teal’s research has enabled us to go beyond broad biological age measurement by looking deeply into organ-specific age profiles. We can now use this technology to understand how individual organ systems are uniquely aging. This information has been shown to be useful in predicting risk and onset of organ-specific diseases like cardiovascular disease, kidney disease, Alzheimer’s, and more. This enables us to create personalized risk profiles based on an individual’s organ aging signature, unlocking personalized health risk assessments and granular stratification, such as brain agers versus heart agers.
There is plenty more to unpack from this work and we will continue to present our approach and findings in additional detail in future articles. For now, we feel a strong responsibility to bring this technology to the world and believe that this approach promises to accelerate our ability to deliver impactful interventions for extending health span.
The odyssey has just begun, and the road from detection to prevention is expected to be wrought with major challenges. But we trust that the field will fight its way toward novel breakthroughs that will grant people the dignity they deserve: to savor the later stages of life unencumbered by disease.
Endnotes
1. GHE: Life expectancy and healthy life expectancy. (n.d.). https://www.who.int/data/gho/data/themes/mortality-and-global-health-estimates/ghe-life-expectancy-and-healthy-life-expectancy#:~:text=Globally%2C%20life%20expectancy%20has%20increased,reduced%20years%20lived%20with%20disability.
2. Bocklandt, S., Lin, W., Sehl, M. E., Sánchez, F. J., Sinsheimer, J. S., Horvath, S., & Vilain, É. (2011). Epigenetic predictor of age. PLOS ONE, 6(6), e14821. https://doi.org/10.1371/journal.pone.0014821
3. Horvath, S. (2013). DNA methylation age of human tissues and cell types. GenomeBiology.com (London. Print), 14(10), R115. https://doi.org/10.1186/gb-2013-14-10-r115
4. Hannum, G., Guinney, J., Zhao, L., Zhang, L., Hughes, G., Sadda, S. V. R., Klotzle, B., Bibikova, M., Fan, J., Gao, Y., Deconde, R., Chen, M., Rajapakse, I., Friend, S. H., Ideker, T., & Zhang, K. (2013). Genome-wide methylation profiles reveal quantitative views of human aging rates. Molecular Cell, 49(2), 359–367. https://doi.org/10.1016/j.molcel.2012.10.016
5. Levine, M. E., Lu, A. T., Quach, A., Chen, B. H., Assimes, T. L., Bandinelli, S., Hou, L., Baccarelli, A. A., Stewart, J. D., Li, Y., Whitsel, E. A., Wilson, J. G., Reiner, A. P., Aviv, A., Lohman, K., Liu, Y., Ferrucci, L., & Horvath, S. (2018). An epigenetic biomarker of aging for lifespan and healthspan. Aging, 10(4), 573–591. https://doi.org/10.18632/aging.101414
6. Lu, A. T., Quach, A., Wilson, J. G., Reiner, A. P., Aviv, A., Raj, K., Hou, L., Baccarelli, A. A., Li, Y., Stewart, J. D., Whitsel, E. A., Assimes, T. L., Ferrucci, L., & Horvath, S. (2019). DNA methylation GrimAge strongly predicts lifespan and healthspan. Aging, 11(2), 303–327. https://doi.org/10.18632/aging.101684
7. Belsky, D. W., Caspi, A., Corcoran, D. L., Sugden, K., Poulton, R., Arseneault, L., Baccarelli, A. A., Chamarti, K., Gao, X., Hannon, E., Harrington, H. L., Houts, R., Kothari, M., Kwon, D., Mill, J., Schwartz, J., Vokonas, P., Wang, C., Williams, B., & Moffitt, T. E. (2022). DunedinPACE, a DNA methylation biomarker of the pace of aging. eLife, 11. https://doi.org/10.7554/elife.73420
8. Bell, C. G., Lowe, R., Adams, P. D., Baccarelli, A. A., Beck, S., Bell, J. T., Christensen, B. C., Gladyshev, V. N., Heijmans, B. T., Horvath, S., Ideker, T., Issa, J. J., Kelsey, K. T., Marioni, R. E., Reik, W., Relton, C. L., Schalkwyk, L. C., Teschendorff, A. E., Wagner, W., . . . Rakyan, V. K. (2019). DNA methylation aging clocks: challenges and recommendations. Genome Biology, 20(1). https://doi.org/10.1186/s13059-019-1824-y
9. Levine, M. E., Higgins‐Chen, A., Thrush, K., Minteer, C. J., & Niimi, P. (2022). Clock Work: Deconstructing the epigenetic clock signals in aging, disease, and reprogramming. bioRxiv (Cold Spring Harbor Laboratory). https://doi.org/10.1101/2022.02.13.480245
10. Higgins‐Chen, A., Thrush, K., Wang, Y., Minteer, C. J., Kuo, P., Wang, M., Niimi, P., Sturm, G., Lin, J., Moore, A. Z., Bandinelli, S., Vinkers, C. H., Vermetten, E., Rutten, B. P. F., Geuze, E., Okhuijsen-Pfeifer, C., Van Der Horst, M. Z., Schreiter, S., Gutwinski, S., . . . Levine, M. E. (2022). A computational solution for bolstering reliability of epigenetic clocks: implications for clinical trials and longitudinal tracking. Nature Aging, 2(7), 644–661. https://doi.org/10.1038/s43587-022-00248-2
11. Sillanpää, E., Heikkinen, A., Kankaanpää, A., Paavilainen, A., Kujala, U. M., Tammelin, T., Kovanen, V., Sipilä, S., Pietiläinen, K. H., Kaprio, J., Ollikainen, M., & Laakkonen, E. K. (2021). Blood and skeletal muscle ageing determined by epigenetic clocks and their associations with physical activity and functioning. Clinical Epigenetics, 13(1). https://doi.org/10.1186/s13148-021-01094-6
12. Föhr, T., Törmäkangas, T., Lankila, H., Viljanen, A., Rantanen, T., Ollikainen, M., Kaprio, J., & Sillanpää, E. (2021). The Association between Epigenetic clocks and physical functioning in Older Women: A 3-Year Follow-up. The Journals of Gerontology, 77(8), 1569–1576. https://doi.org/10.1093/gerona/glab270
13. Kabacik, S., Lowe, D., Fransen, L. F. H., Leonard, M. O., Ang, S., Whiteman, C., Corsi, S., Cohen, H. A., Felton, S., Bali, R., Horvath, S., & Raj, K. (2022). The relationship between epigenetic age and the hallmarks of aging in human cells. Nature Aging, 2(6), 484–493. https://doi.org/10.1038/s43587-022-00220-0
14. Olova, N., Simpson, D. J., Marioni, R. E., & Chandra, T. (2018). Partial reprogramming induces a steady decline in epigenetic age before loss of somatic identity. Aging Cell, 18(1), e12877. https://doi.org/10.1111/acel.12877
15. Sarkar, T. J., Quarta, M., Mukherjee, S., Colville, A., Paine, P., Doan, L., Tran, C., Chu, C. R., Horvath, S., Qi, L. S., Bhutani, N., Rando, T. A., & Sebastiano, V. (2020). Transient non-integrative expression of nuclear reprogramming factors promotes multifaceted amelioration of aging in human cells. Nature Communications, 11(1). https://doi.org/10.1038/s41467-020-15174-3
16. Villeda, S., Plambeck, K. E., Middeldorp, J., Castellano, J. M., Mosher, K. I., Luo, J., Smith, L. K., Bieri, G., Lin, K., Berdnik, D., Wabl, R., Udeochu, J. C., Wheatley, E., Zou, B., Simmons, D. A., Xie, X., Longo, F. M., & Wyss‐Coray, T. (2014). Young blood reverses age-related impairments in cognitive function and synaptic plasticity in mice. Nature Medicine, 20(6), 659–663. https://doi.org/10.1038/nm.3569